Research
Chromosome replication is performed by a large multisubunit assembly, known as the replisome, which couples the unpackaging of parental DNA with the synthesis of new daughter strands.
Chromosome replication is performed by a large multisubunit assembly, known as the replisome, which couples the unpackaging of parental DNA with the synthesis of new daughter strands. To ensure genome integrity, this sophisticated molecular machine must coordinate events over a broad range of time and length scales, from the breaking and reformation of chemical bonds within DNA polymerases to the large-scale structural rearrangements of chromosomes. We seek to define the operating principles that guide replisome function and understand how replisomes recognize and process obstacles encountered on parental chromosomes such as DNA lesions, unfavorable topological structures, and architectural factors.
Our vision is to use these studies to develop a general framework for understanding how networks of intra- and inter-molecular interactions can evolve to guide dynamic events within multiprotein complexes.
Chromatin Replication
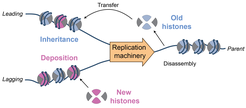
The storage and transmission of genetic information is a fundamental challenge faced by all cellular organisms. Chromosomes must be folded and compacted in an orderly fashion to fit inside cells but retain dynamic flexibility to allow for rapid information access. These intricate structures must likewise allow for efficient disassembly and reassembly during replication.
In eukaryotic organisms, nucleosomes function as the most basic unit of chromosome organization directly binding and assembling on DNA to modulate DNA topology, drive compaction, and regulate transcription. They consist of a dimer of dimers with two copies each of the histones H3, H4, H2A and H2B, that tightly bind and wrap DNA, while leaving long exposed tails available for post-translational modification that can carry epigenetic information. This basic unit is modulated by a myriad of chaperones and remodellers that modify, reposition, and restructure nucleosomes to accomplish various essential chromosomal functions.
The dynamic events that underlie the disassembly and reassembly of nucleosomes during replication remains a long-standing puzzle in chromosome biology. Nucleosomes pose a two-fold problem for the replication machinery. They must be removed to allow access to the genetic information. However, parental histones must also be transferred to the daughter-strands to ensure epigentic information is faithfully replicated. Moreover, this inheritance pathway must be coordinated with the deposition of newly synthesized histones to ensure chromosome duplication.
In a major new project, ReplisomeBypass, funded by a European Research Council (ERC) Starting Grant, we are developing single-molecule approaches to directly visualize replisome dynamics during nucleosome encounters. By reconstituting the S. Cerevisiae replication machinery at the single complex level we are able to study critical transient intermediates formed during chromatin replication. These unique experimental approaches will clarify the distinct roles of key factors in coordinating inheritance and deposition.
Mechanics of Topological Rearrangements during DNA Replication
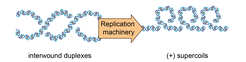
While the double-helical structure of duplex DNA is advantageous for the storage and maintenance of genetic information, it poses major challenges during essential cellular processes, such as transcription and replication, when the information-rich DNA bases must be accessed. Separation of the two single strands leads to the build-up of superhelical tension, which, if left unresolved, exerts extreme forces in the form of torque that disrupts critical enzymatic events on chromosomes.
During replication, separation of the two daughter-strand templates by the helicase leads to overwinding and the formation of positive supercoils ahead of the replication fork. Likewise, superhelical tension forms on the daughter strands as a consequence of polymerase synthesis. Polymerases must travel on a helical path during successive rounds of nucleotide incorporation to generate a helical, double-stranded DNA product. Since the physical tethering of the polymerases within the replisome holds them in fixed orientations, the daughter strands must rotate to accommodate the path of synthesis.
Resolution of superhelical tension is critical to ensure replication fork progression and avoid chromosome damage. To date, studies of this important problem have been focused on topoisomerases, which are a specialized class of enzymes that can resolve superhelical tension. Studies of these factors have proven tremendously fruitful yielding several classes of potent antibiotics and anticancer drugs (e.g. ciprofloxacin and camptothecin). However, the other side of the problem – namely, how replisomes operate when faced with topological stress – has remained largely unexplored. Replisomes must possess some resistance to topological stress given the dynamics at rapidly moving replication forks, near chromosome ends, and under moderate conditions of inhibition, where topoisomerases struggle to keep pace with fork progression.
In a project supported by the collaborative research center Forces in Biomolecular Systems (SFB 863), we are developing a single-molecule nanomanipulation platform to study replisome dynamics under topological stress conditions. These studies will clarify the intrinsic ability of the replisome to cope with torsional strain, the effect of torsional strain on replisome integrity, and fork rotation dynamics.
Single Molecule Imaging
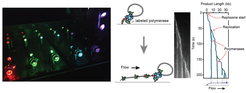
Classical biochemical methods have provided tremendous insight into the basic factors and mechanisms of DNA replication. However, these methods are fundamentally limited by ensemble averaging, which obscures differences within a population. Gaining access to a more detailed molecular understanding of replisome dynamics depends on the development and implementation of novel imaging approaches.
We employ cutting-edge single-molecule imaging approaches that provide high spatial and temporal resolution to study the dynamics of single replisomes in real-time. To study the coordination of daughter-strand synthesis, we use a multidimensional DNA flow-stretching assay in which a flow through a flowcell applies a force on individual surface immobilized DNA substrates. We then directly track replication fork progression either using micron-sized beads attached at the DNA ends or by fluorescence staining. Using co-localization single-molecule spectroscopy with fluorescently labeled replisome components reveals the distribution of individual factors in and around the replication fork as well as their exchange dynamics.