Research
Our laboratory works to understand the molecular mechanisms at play in major eukaryotic RNA pathways. We use cutting-edge biochemical and structural biology approaches to elucidate the structures of protein-RNA complexes and we contextualize the atomic and chemical information in the framework of cellular function and biological significance.
RNAs are fundamental molecules of life. They consist of ribonucleotide chains that exist in different sizes and shapes, as they can fold and adopt intricate three-dimensional configurations. This diversity parallels the different roles that RNAs have in our cells, impacting both healthy and disease states. A particularly important type of RNA is messenger RNA (mRNA). mRNAs are the temporary go-betweens that carry the copy of a gene to the protein synthesis machinery. In eukaryotic cells, transcription of DNA to mRNA occurs in the nucleus, while translation of mRNAs into proteins occurs in the cytoplasm. Eukaryotic mRNAs therefore have to be assembled and then transported from one cellular compartment to the other. The cytoplasm is where the rather brief life of an mRNA generally ends, as mRNAs have to be degraded once the cellular demand for the corresponding protein has ceased.
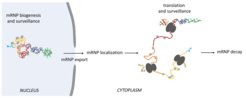
A major focus of our laboratory is to unravel the molecular mechanisms responsible for the controlled degradation of mRNA molecules. In addition to regulating protein production according to to the ‘supply and demand’ of the cell, mRNA degradation also plays crucial roles in the elimination of non-productive or defective transcripts. This line of investigation has broad significance due to the many genetic diseases associated with the protein complexes involved in mRNA clearance, ranging from blood disorders to neurological diseases. Conversely, we are also intrigued by the mechanisms with which productive mRNAs are packaged, safeguarded and transported through the crowded cellular environment to reach the protein-synthesis machineries. Events that are only, further complicated by the fact that mRNAs do not exist in isolation but in the context of mRNA-protein complexes, forming messenger ribonucleoprotein particles (mRNPs). Despite the discovery of mRNAs over a half century ago, little is known about the composition and architecture of individual mRNPs, and how changes in mRNP structure relate to their function or to dysfunction. Answering these questions is another major focus of our laboratory. We believe that understanding the structure and properties of mRNPs and of the molecular machines that interact with them holds the key to unraveling the essential roles they play in many cellular processes.
To gain mechanistic insights into the assembly and function of protein-RNA complexes at the molecular and chemical level, we first purify the macromolecular complexes either from endogenous sources or by biochemical reconstitution using recombinant proteins and in vitro transcribed RNAs. We then subject them to an in-depth characterization that encompasses various techniques (such as in vitro assays, RNA sequencing, mass spectrometry analysis, biophysical measurements) and then decipher their architecture using an integrated structural biology approach that includes a combination of cryo-EM/tomography, X-ray crystallography, crosslinking mass spectrometry and predictive machine learning software. Last but not least, we assess the physiological relevance of our findings by in vivo studies (in yeast, human cells or through collaborations in other model systems).
As a whole, over the past 25 years, our group has been at the forefront of advancing protein-RNA biochemistry and incorporating cutting-edge developments in structural biology to address the biological questions that drive our research, and we remain steadfast in our determination to progress along this upward trajectory.

Research Questions:
The consensual view of cytoplasmic mRNA turnover is that of a sequential process that starts with the remodeling and shortening of the 3’ end poly(A) tail (via the Pan2-Pan3 and Ccr4-Not deadenylases) and ends with the degradation of the mRNA body, either in the 3-5’ direction (by the exosome and its cofactors) or in the 5’-3’ direction (by removal of the cap structure and 5’-3’ degradation by Xrn1). We have been working to understand the molecular mechanisms of the Pan2-Pan3 and Ccr4-Not deadenylases and the RNA exosome complexes. A current focus is to understand the impact of the poly(A) RNP on the initial trimming steps, as this greatly influences degradation overall. Another major line of inquiry is to understand the mechanisms with which ribosome-bound mRNAs are targeted for exosome-mediated degradation. The recruitment of the exosome is achieved by an additional complex (known as Ski, for Superkiller) that we recently found undergoes a series of conformational changes to thread RNA through to the exosome. Altogether, we have unveiled unexpected regulatory mechanisms that are converging to form a unified picture of controlled degradation as a feature conserved between lower and higher eukaryotes.
RNA degradation has a pivotal role in quality-control pathways that detect and eliminate defective mRNAs. Our laboratory has been working over the years on the mechanisms of nonsense-mediated mRNA decay (NMD), a surveillance pathway that eliminates mRNAs with premature termination codons (PTCs) in addition to regulating parts of the transcriptome. Arguably, the central, evolutionary conserved factor in NMD is the UPF1 protein. UPF1 is an RNA helicase protein that is activated upon phosphorylation. In yeast, Upf1 is found as part of two mutually exclusive complexes that are believed to represent different steps in the NMD pathway. In human cells, the situation is considerably more complex, as more proteins come into play.
An important factor in human cells is the exon junction complex (EJC), an assembly of proteins that is deposited on mRNAs in the nucleus upon splicing and is exported as part of the spliced mRNP in the cytoplasm. Additionally, another set of factors participates in either phosphorylating UPF1 (SMG1, SMG8, SMG9) or recognizing its phosphorylation state (SMG5, SMG6 and SMG7). We have been elucidating the interactions that connect UPF1 to the EJC and unraveled the molecular mechanisms involved in UPF1 phosphorylation and its subsequent detection within the NMD pathway. Current efforts are aimed at understanding the interplay between different human UPF1-containing complexes in the context of a premature translation-termination event, i.e., to decipher the chain of events that initiate with a ribosome stalled at a PTC and culminate in the degradation of the PTC-containing mRNA.
While the nucleus is often seen as the control center of the cell, recently, the nuclear compartment has emerged as a very active environment for RNA degradation. Here, RNAs are transcribed as precursors and undergo various degradative actions that result in either the productive trimming of the precursors to their mature form or the elimination of transcripts originating from abortive transcription or faulty processing. The RNA-degrading exosome is a key player in all these processes. Using cryo-EM, we now understand how the nuclear exosome processes RNA in the maturation of the large ribosomal subunit, i.e., how the degradation machinery can be repurposed to guide the ribosomal RNP to a productive pathway. The major functions of nuclear exosomes, however, are in destructive pathways, such as the elimination of nonfunctional, noncoding RNAs that arise from faulty polymerase (Pol) II transcription. These transcripts are particularly abudant in human cells, which indeed have an extensive network of nuclear exosome cofactors dedicated to the purpose of removal. In particular, the NEXT (Nuclear EXosome Targeting) and PAXT (Poly(A) tail eXosome Targeting) exosome cofactor complexes operate in the context of proteins that are important for the nuclear export of mature mRNPs (such as the 5’ cap-binding complex and the nuclear poly(A)-binding protein PABPN1). Our current efforts are focused on deciphering the mechanisms that discriminate and guide the productive and destructive pathways of capped and polyadenylated Pol II transcripts.
While in the past years we have focussed primarily on trying to understand how and why faulty RNAs are eliminated, we are now addressing the opposite question: what makes a Pol II transcript a mature, export-competent mRNP? Although it is known that a transcript has to undergo proper transcription termination with the addition of the poly(A) tail and poly(A)-binding proteins (PABPN1 in humans, Nab2 in yeast), this process is still not completely understood. Furthermore, the construction of an mRNP begins at an even earlier stage—as the mRNA is being transcribed and modified. Currently, our group is engaged in developing methods to purify endogenous mRNP populations from either yeast or human cells with the goal of eventually understanding how they are built. We plan to further adapt these methods to study mRNPs in a developmental setting, such as translationally-repressed mRNPs in Drosophila embryos. In general, we believe that understanding how our cells package and safeguard their own mRNAs is not only a crucial question for understanding gene expression programs, but also has potentially far-reaching implications given the rapid developments of mRNA-based therapeutics.