Ångström-Resolution Fluorescence Microscopy
Research Team achieves Ångström Resolution using DNA-barcoded Fluorescence Microscopy
A breakthrough in fluorescence microscopy has been achieved by the research group of Ralf Jungmann at the Max Planck Institute of Biochemistry (MPIB) and Ludwig-Maximilians-Universität (LMU) Munich. The team developed Resolution Enhancement by Sequential Imaging (RESI), a revolutionary technique that enhances the resolution of fluorescence microscopy down to the Ångström scale. This innovation is poised to usher in a paradigm shift in our approach to study biological systems with thus far unprecedented detail.
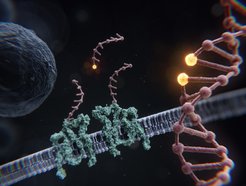
Cells, the fundamental units of life, contain a plethora of intricate structures, processes and mechanisms that uphold and perpetuate living systems. Many cellular core components, such as DNA, RNA, proteins and lipids, are just a few nanometers in size. This makes them substantially smaller than the resolution limit of traditional light microscopy. The exact composition and arrangement of these molecules and structures is thus often unknown, resulting in a lack of mechanistic understanding of fundamental aspects of biology.
In recent years, super-resolution techniques have made leaps and bounds to resolve many sub-cellular structures below the classical diffraction limit of light. Single molecule localization microscopy, or SMLM, is a super-resolution approach that can resolve structures on the order of ten nanometers in size by temporally separating their individual fluorescence emission. As individual targets stochastically light up (they blink) in an otherwise dark field of view, their location can be determined with sub-diffraction precision. DNA-PAINT, invented by the Jungmann group, is a SMLM technique that uses transient hybridization of dye-labeled DNA “imager” strands to their target-bound complements to achieve the necessary blinking for super-resolution. However, to date, even DNA-PAINT has not been able to resolve the smallest cellular structures.
In the current study led by co-first authors Susanne Reinhardt, Luciano Masullo, Isabelle Baudrexel and Philipp Steen together with Jungmann, the team introduces a novel approach in super-resolution microscopy that enables fundamentally “unlimited” spatial resolution. The new technique, called “Resolution Enhancement by Sequential Imaging”, or RESI for short, capitalizes on the ability of DNA-PAINT to encode target identity via unique DNA sequences. By labeling adjacent targets, too close to each other to be resolved even by super-resolution microscopy, with different DNA strands, an additional degree of differentiation (a barcode) is introduced into the sample. By sequentially imaging first one, and then the other sequence (and thereby target), they can now be unambiguously separated. Critically, as they are imaged sequentially, the targets can be arbitrarily close to each other, something no other technique can resolve. Furthermore, RESI does not require specialized instrumentation, in fact, it can be applied using any standard fluorescence microscope, making it easily accessible for almost all researchers.
To demonstrate RESI’s leap in resolution, the team set themselves the challenge of resolving one of the smallest spatial distances in a biological system: The separation between individual bases along a double helix of DNA, spaced less than one nanometer (a billionth of a meter) apart. By designing a DNA origami nanostructure such that it presents single-stranded DNA sequences that protrude from a double helix at one base pair distance and then imaging these single strands sequentially, the research team resolved a distance of 0.85 nm (or 8.5 Ångström) between adjacent bases, a previously unimaginable feat. The researchers accomplished these measurements with a precision of 1 Ångström, or one ten-billionth of a meter, underscoring the unprecedented capabilities of the RESI approach.
Importantly, the technique is universal and not limited to applications in DNA nanostructures. To this end, the team investigated the molecular mode of action of Rituximab, an anti-CD20 monoclonal antibody that was first approved in 1997 for treatment of CD20-positive blood cancer. However, investigating the effects of such drug molecules on molecular receptor patterns has been beyond the spatial resolution capabilities of traditional microscopy techniques. Understanding whether and how such patterns change in health and disease as well as upon treatment is not only important for basic mechanistic research, but also for designing novel targeted disease therapies. Using RESI, Jungmann and his team were able to reveal the natural arrangement of CD20 receptors in untreated cells as dimers and uncover how CD20 re-arranged to chains of dimers upon drug treatment. The insights on the single-protein level now help to shed light on the molecular mode of action of Rituximab.
As RESI is performed in whole, intact cells, the technique closes the gap between purely structural techniques such as X-ray crystallography or cryogenic electron microscopy and traditional lower resolution whole-cell imaging approaches. Jungmann and his team are convinced that “this unprecedented technique is a true game-changer not only for super-resolution, but for biological research as a whole”.
