The importance of interfaces
A team of researchers from Germany and the UK discover new approaches on the origin of life.
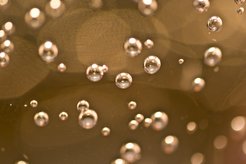
A plethora of physicochemical processes must have created the conditions that enabled living systems to emerge on the early Earth. In other words, the era of biological evolution must have been preceded by a – presumably protracted – phase of ‘prebiotic’ chemical evolution, during which the first informational molecules capable of replicating themselves were assembled and selected. This scenario immediately raises another question: Under what environmental conditions could prebiotic evolution have taken place? One possible setting has long been discussed and explored – tiny pores in volcanic rocks. An international team of researchers, with participation of Hannes Mutschler and Petra Schwille from the Max Planck Institute of Biochemistry and scientists from the LMU Munich and UC London, has now taken a closer look at the water-air interfaces in these pores. They form spontaneously at gas-filled bubbles and show an interesting combination of effects. They found that they could have played an important part in facilitating the physicochemical interactions that contributed to the origin of life. The question was whether such interfaces could have stimulated the kinds of chemical reactions that triggered the initial stages of prebiotic chemical evolution. Their findings appear in the leading journal Nature Chemistry.
The study strongly supports the notion that tiny gas-filled bubbles that were trapped in, and reacted with, the surfaces of pores in volcanic rocks could indeed have accelerated the formation of the chemical networks that ultimately gave rise to the first cells. Thus, the authors were able to experimentally verify and characterize the facilitating effects of air-water interfaces on the relevant chemical reactions. If there is a difference in temperature along the surface of such a bubble, water will tend to evaporate on the warmer side and condense on the cooler side, just as a raindrop that lands on a window runs down the flat surface of the glass and eventually evaporates. This process can be repeated ad infinitum, since the water continuously cycles between the gaseous and the liquid phase. The upshot of this cyclical phenomenon is that molecules accumulate to very high concentrations on the warmer side of the bubble.
This was followed by experiments with a series of physical and chemical processes which must have played a central role in the origin of life – all of them were markedly accelerated or made possible at all under the conditions prevailing at the air-water interface. The phenomenon turned out to be surprisingly effect and robust. Even small molecules could be concentrated to high levels.
For example, the researchers show that physicochemical processes which promote the formation of polymers are either stimulated – or made possible in the first place – by the availability of an interface between the aqueous environment and the gas phase, which markedly enhances rates of chemical reactions and catalytic mechanisms. In fact, in such experiments, molecules could be accumulated to high concentrations within lipid membranes, insoluble in water, when the researchers added the appropriate chemical constituents. The vesicles, cell bubbles, produced in this way are not perfect. But the finding nevertheless suggests how the first rudimentary protocells and their outer membranes might have been formed.
Whether or not this sort of process can take place in such vesicles does not depend on the nature of the gas within the bubble. What is important is that, owing to differences in temperature, the water can evaporate in one location and condense in another.
"This unique mechanism makes it possible to combine the fundamental processes of RNA generation, RNA catalysis and RNA encapsulation in one place" explains Hannes Mutschler, head of the research group "Biomimetic Systems" at the Max Planck Institute of Biochemistry.
Original publication:
M. Morasch, J. Liu, CF. Dirscherl, A. Ianeselli, A. Kühnlein, K. L. Vay, P. Schwintek, S. Islam, MK. Corpinot, B. Scheu, DB. Dingwell, P. Schwille, H. Mutschler, M. W. Powner, C. B. Mast & D. Braun. Heated gas bubbles enrich, crystallize, dry, phosphorylate and encapsulate prebiotic molecules. Nature, Juli 2019
DOI: https://doi.org/10.1038/s41557-019-0299-5