Phototaxis in Halobacterium: A testcase for systems biology
The phototaxis of Halobacterium is analyzed in a joint project of
Wolfgang Marwan, MPI for Dynamics of Complex Technical Systems, Magdeburg
E.D. Gilles, MPI for Dynamics of Complex Technical Systems, Magdeburg
Dieter Oesterhelt, MPI of Biochemistry, Dept. Membrane Biochemistry, Martinsried
A major contribution was made by T. Nutsch (PhD thesis in the group of E.D. Gilles).
Using the photophobic response mediating phototaxis of Halobacterium salinarum as example, the poster illustrates how interactions at different levels of complexity (photon, small molecule, protein complex, network of interacting proteins, protein supercomplex, living cell) cooperatively control the intelligent behaviour of a living cell. At the same time it shows that these molecular events can be observed, measured, and described at the system level by mathematical models. In such models, all biochemical reactions are integrated and the overall process is simulated in the computer. Such simulations permit to view the functioning of the whole system (assuming that the model is correct) and help to identify residual problems resulting from an incomplete or incorrect understanding of the system.
H. salinarum actively searches for the optimal place to live. It can use (orange) light as energy source but since its DNA is damaged by UV light, Halobacterium has developed a color vision system to measure light intensity at different wavelengths and evaluate the pros and cons of the ambient light composition to find the appropriate place to stay. (In addition, it can scan for oxygen, various chemicals, and additional yet unidentified external signals). As a motile organism, Halobacterium actively moves towards attractants and flees from repellents (by a technique called "biased random walk").
-
phototaxis: tactic response to light
-
chemotaxis: tactic response to chemicals, e.g. nutrients
-
aerotaxis: tactic response to oxygen
(see also the Introduction to the tactic response).
The photophobic response of Halobacterium salinarum has been analyzed in detail (e.g. by motion analysis, protein characterization, spectroscopy) and a large amount of quantitative data have been collected. Thus, this process is an excellent model for application of systems biology tools.
(Row 1)
A behavioural response of a cell can be triggered by a simple physical stimulus (a photon of light) absorbed by a small molecule, retinal (vitamine A aldehyde), which is covalently attached to the photoreceptor protein sensory rhodopsin I. Retinal has a dual function as light antenna to capture the photon and as a light-activated switch: Photoexcitation makes retinal to change its conformation by isomerization and to deliver this steric trigger to the protein moiety of sensory rhodopsin I. The protein moiety has a dual function as well. It relays the signal to the cognate transducer molecule and it reisomerizes retinal to regenerate its initial, light-sensitive state.
(Rows 2 and 3)
The transducer of sensory rhodopsin relays its activation state to the biochemical network that controls the flagellar motor. Receptor activation and conformational coupling with the transducer is understood at the atomic level for the orthologous protein complex from Natronomonas pharaonis (Gordeliy et al., 2002).
(Row 4)
The photoreceptor/transducer complex interacts with a biochemical network centered around a central "two-component regulator" (consisting of two major proteins: CheA and CheY). The output of this network is the concentration of phosphorylated CheY. Within the network, the following signal tranduction steps take place:
-
"amplification": the signal is amplified
-
"integration": signals from various stimuli are integrated
-
"adaptation": return of the signalling system to the pre-stimulus level while the stimulus persists
(Row 5)
The flagellar motor is the target of the signaling pathway. The functional mechanism of this molecular maschine and the mode of interaction of its subunits have been elucidated. This was possible without identifying its biochemical components. The model has predictive power: it suggests that the subunits of the switch complex of the flagellar motor do not only switch the sense of motor rotation, but in addition actively contribute to processing of the input signal transmitted through the two-component system.
(Row 6)
A detailed mathematical model of the flagellar motor has been developed (Nutsch et al., 2005). Due to the high density of quantitative data for the phototactic response of Halobacterium, the predictions from the model could be compared to the experimental data and to this end proved to explain all of them.
Systems biology:
Molecular interactions at different levels of molecular complexity determine the behaviour of a living cell
The energy of a single photon (E = h ν) can trigger behavioral response
Levels of complexity:

Photon captured by retinal, a dye of low molecular weight
Sensory rhodopsin-transducer complex
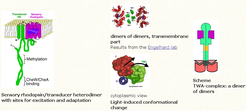
Biochemical Network
connects receptors to the flagellar motor and regulates the response of the cell
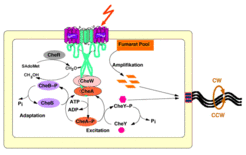
Archaeal Flagellar Motor:

macromolecular complex and signal processing maschine
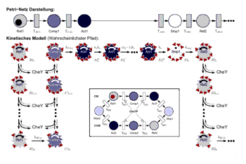
Behavioural response:
of the living cell as predicted by the mathematical model

References:
-
Gordeliy VI, Labahn J, Moukhametzianov R, Efremov R, Granzin J, Schlesinger R, Buldt G, Savopol T, Scheidig AJ, Klare JP, Engelhard M.
Molecular basis of transmembrane signalling by sensory rhodopsin II-transducer complex.
Nature 419: 484-487 (2002) - T. Nutsch, D. Oesterhelt, E.D. Gilles and W. Marwan:
The Switch Cycle of an Archaeal Flagellar Motor and its Sensory Control.
Biophys. J. 89, 2307-2323 (2005).